What Kind of Product is the Supercapacitor Structure?
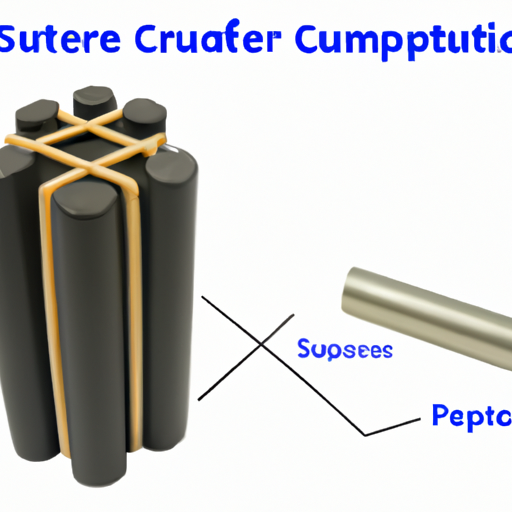
I. Introduction
In an era where energy efficiency and sustainability are paramount, the quest for effective energy storage solutions has never been more critical. Among the various technologies available, supercapacitors have emerged as a compelling option. This article aims to explore the structure, functionality, and applications of supercapacitors, shedding light on their significance in the modern energy landscape.
II. Understanding Supercapacitors
A. Basic Principles of Supercapacitors
Supercapacitors, also known as ultracapacitors or electrochemical capacitors, are energy storage devices that store energy through electrostatic charge separation. Unlike traditional capacitors that store energy in an electric field, supercapacitors utilize the electrostatic charge at the interface between an electrode and an electrolyte. This unique mechanism allows them to achieve higher energy storage capacities compared to conventional capacitors.
When compared to batteries, supercapacitors offer distinct advantages. While batteries rely on chemical reactions to store and release energy, supercapacitors can charge and discharge much faster, making them ideal for applications requiring rapid bursts of energy.
B. Components of a Supercapacitor
A supercapacitor consists of three primary components:
1. **Electrodes**: These are the conductive materials that store charge. The performance of a supercapacitor largely depends on the materials used for the electrodes.
2. **Electrolyte**: This medium facilitates the movement of ions between the electrodes during charging and discharging. The choice of electrolyte can significantly influence the supercapacitor's performance.
3. **Separator**: This component prevents direct contact between the electrodes while allowing ionic movement, ensuring safe and efficient operation.
C. Types of Supercapacitors
Supercapacitors can be categorized into three main types:
1. **Electric Double-Layer Capacitors (EDLCs)**: These utilize electrostatic charge separation at the electrode-electrolyte interface, offering high power density and long cycle life.
2. **Pseudocapacitors**: These employ fast redox reactions at the electrode surface, providing higher energy density than EDLCs but typically at the cost of cycle life.
3. **Hybrid Capacitors**: These combine features of both EDLCs and batteries, aiming to achieve a balance between energy and power density.
III. The Structure of Supercapacitors
A. Design and Materials
The structure of supercapacitors is crucial for their performance. Key design elements include:
1. **Electrode Materials**:
- **Carbon-based Materials**: Activated carbon is the most common material due to its high surface area and conductivity.
- **Conductive Polymers**: These materials can enhance energy density and are often used in pseudocapacitors.
- **Metal Oxides**: These can provide high capacitance but may have limitations in terms of conductivity and cycle life.
2. **Electrolyte Types**:
- **Aqueous Electrolytes**: These are safe and cost-effective but have lower voltage limits.
- **Organic Electrolytes**: These allow for higher voltage operation but can be more expensive and less environmentally friendly.
- **Ionic Liquids**: These offer a wide electrochemical window and high thermal stability, making them suitable for advanced applications.
3. **Separator Materials**: The separator must be porous enough to allow ion movement while preventing electrical contact between the electrodes. Common materials include cellulose, polypropylene, and glass fiber.
B. Manufacturing Processes
The production of supercapacitors involves several key processes:
1. **Synthesis of Materials**: This includes the preparation of electrode materials, electrolytes, and separators, often requiring advanced techniques to ensure high purity and performance.
2. **Assembly Techniques**: The assembly of supercapacitors must be done in controlled environments to prevent contamination and ensure optimal performance.
3. **Quality Control Measures**: Rigorous testing is essential to ensure that the supercapacitors meet performance specifications and safety standards.
IV. Performance Characteristics
Supercapacitors are characterized by several performance metrics:
A. Energy Density vs. Power Density
Energy density refers to the amount of energy stored per unit volume or mass, while power density indicates how quickly energy can be delivered. Supercapacitors typically have lower energy density compared to batteries but excel in power density, making them suitable for applications requiring quick bursts of energy.
B. Charge and Discharge Rates
Supercapacitors can charge and discharge in seconds, significantly faster than batteries. This rapid response time is crucial for applications like regenerative braking in electric vehicles and power backup systems.
C. Cycle Life and Durability
One of the standout features of supercapacitors is their long cycle life. They can endure hundreds of thousands to millions of charge-discharge cycles without significant degradation, making them ideal for applications where longevity is essential.
D. Temperature Stability
Supercapacitors can operate effectively across a wide temperature range, which is advantageous for various applications, from consumer electronics to industrial systems.
V. Applications of Supercapacitors
Supercapacitors find applications across a diverse range of sectors:
A. Consumer Electronics
In devices like smartphones and laptops, supercapacitors can provide quick bursts of power, enhancing performance during peak usage.
B. Electric Vehicles
Supercapacitors are increasingly used in electric vehicles for regenerative braking systems, allowing for rapid energy recovery and improved efficiency.
C. Renewable Energy Systems
In solar and wind energy systems, supercapacitors can store excess energy generated during peak production times, providing a buffer for energy supply.
D. Industrial Applications
Supercapacitors are used in various industrial applications, including uninterruptible power supplies (UPS) and backup power systems, where reliability and quick response times are critical.
E. Emerging Technologies
As technology advances, supercapacitors are being integrated into new applications, such as smart grids and IoT devices, where efficient energy management is essential.
VI. Advantages and Limitations
A. Advantages of Supercapacitors
1. **Fast Charging and Discharging**: Supercapacitors can be charged and discharged in seconds, making them ideal for applications requiring rapid energy delivery.
2. **Long Cycle Life**: With the ability to withstand millions of cycles, supercapacitors offer a longer lifespan compared to traditional batteries.
3. **Wide Temperature Range**: Their ability to operate effectively in various temperatures makes them versatile for different environments.
B. Limitations
1. **Lower Energy Density Compared to Batteries**: While supercapacitors excel in power density, their energy density is lower than that of batteries, limiting their use in applications requiring long-term energy storage.
2. **Cost Considerations**: The manufacturing costs of high-performance supercapacitors can be higher than traditional batteries, which may hinder widespread adoption.
3. **Environmental Impact**: The production and disposal of certain materials used in supercapacitors can raise environmental concerns, necessitating sustainable practices.
VII. Future Trends and Innovations
A. Research and Development Directions
Ongoing research aims to enhance the energy density and reduce the costs of supercapacitors, making them more competitive with traditional energy storage solutions.
B. Potential for New Materials
Innovations in materials science, such as the development of nanomaterials and advanced composites, hold promise for improving supercapacitor performance.
C. Integration with Other Energy Storage Technologies
The future may see supercapacitors being integrated with batteries and other energy storage technologies to create hybrid systems that leverage the strengths of each.
D. Market Growth and Economic Impact
As the demand for efficient energy storage solutions continues to rise, the supercapacitor market is expected to grow significantly, impacting various industries and driving economic development.
VIII. Conclusion
Supercapacitors represent a vital component of the future energy landscape, offering unique advantages in energy storage and delivery. Their ability to charge and discharge rapidly, coupled with a long cycle life, positions them as a key player in various applications, from consumer electronics to renewable energy systems. As research and development continue to advance, supercapacitors will likely play an increasingly important role in shaping sustainable energy solutions for the future.
IX. References
- Academic Journals
- Industry Reports
- Books and Articles on Energy Storage Technologies
In summary, supercapacitors are not just a niche product; they are a transformative technology that can enhance energy efficiency and sustainability across multiple sectors. Their unique structure and performance characteristics make them an essential part of the evolving energy landscape.
What Kind of Product is the Supercapacitor Structure?
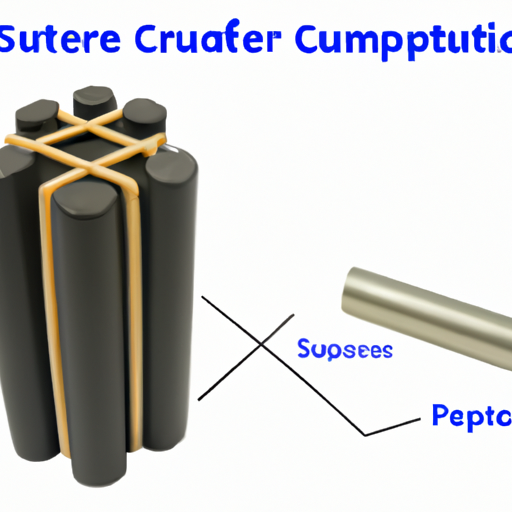
I. Introduction
In an era where energy efficiency and sustainability are paramount, the quest for effective energy storage solutions has never been more critical. Among the various technologies available, supercapacitors have emerged as a compelling option. This article aims to explore the structure, functionality, and applications of supercapacitors, shedding light on their significance in the modern energy landscape.
II. Understanding Supercapacitors
A. Basic Principles of Supercapacitors
Supercapacitors, also known as ultracapacitors or electrochemical capacitors, are energy storage devices that store energy through electrostatic charge separation. Unlike traditional capacitors that store energy in an electric field, supercapacitors utilize the electrostatic charge at the interface between an electrode and an electrolyte. This unique mechanism allows them to achieve higher energy storage capacities compared to conventional capacitors.
When compared to batteries, supercapacitors offer distinct advantages. While batteries rely on chemical reactions to store and release energy, supercapacitors can charge and discharge much faster, making them ideal for applications requiring rapid bursts of energy.
B. Components of a Supercapacitor
A supercapacitor consists of three primary components:
1. **Electrodes**: These are the conductive materials that store charge. The performance of a supercapacitor largely depends on the materials used for the electrodes.
2. **Electrolyte**: This medium facilitates the movement of ions between the electrodes during charging and discharging. The choice of electrolyte can significantly influence the supercapacitor's performance.
3. **Separator**: This component prevents direct contact between the electrodes while allowing ionic movement, ensuring safe and efficient operation.
C. Types of Supercapacitors
Supercapacitors can be categorized into three main types:
1. **Electric Double-Layer Capacitors (EDLCs)**: These utilize electrostatic charge separation at the electrode-electrolyte interface, offering high power density and long cycle life.
2. **Pseudocapacitors**: These employ fast redox reactions at the electrode surface, providing higher energy density than EDLCs but typically at the cost of cycle life.
3. **Hybrid Capacitors**: These combine features of both EDLCs and batteries, aiming to achieve a balance between energy and power density.
III. The Structure of Supercapacitors
A. Design and Materials
The structure of supercapacitors is crucial for their performance. Key design elements include:
1. **Electrode Materials**:
- **Carbon-based Materials**: Activated carbon is the most common material due to its high surface area and conductivity.
- **Conductive Polymers**: These materials can enhance energy density and are often used in pseudocapacitors.
- **Metal Oxides**: These can provide high capacitance but may have limitations in terms of conductivity and cycle life.
2. **Electrolyte Types**:
- **Aqueous Electrolytes**: These are safe and cost-effective but have lower voltage limits.
- **Organic Electrolytes**: These allow for higher voltage operation but can be more expensive and less environmentally friendly.
- **Ionic Liquids**: These offer a wide electrochemical window and high thermal stability, making them suitable for advanced applications.
3. **Separator Materials**: The separator must be porous enough to allow ion movement while preventing electrical contact between the electrodes. Common materials include cellulose, polypropylene, and glass fiber.
B. Manufacturing Processes
The production of supercapacitors involves several key processes:
1. **Synthesis of Materials**: This includes the preparation of electrode materials, electrolytes, and separators, often requiring advanced techniques to ensure high purity and performance.
2. **Assembly Techniques**: The assembly of supercapacitors must be done in controlled environments to prevent contamination and ensure optimal performance.
3. **Quality Control Measures**: Rigorous testing is essential to ensure that the supercapacitors meet performance specifications and safety standards.
IV. Performance Characteristics
Supercapacitors are characterized by several performance metrics:
A. Energy Density vs. Power Density
Energy density refers to the amount of energy stored per unit volume or mass, while power density indicates how quickly energy can be delivered. Supercapacitors typically have lower energy density compared to batteries but excel in power density, making them suitable for applications requiring quick bursts of energy.
B. Charge and Discharge Rates
Supercapacitors can charge and discharge in seconds, significantly faster than batteries. This rapid response time is crucial for applications like regenerative braking in electric vehicles and power backup systems.
C. Cycle Life and Durability
One of the standout features of supercapacitors is their long cycle life. They can endure hundreds of thousands to millions of charge-discharge cycles without significant degradation, making them ideal for applications where longevity is essential.
D. Temperature Stability
Supercapacitors can operate effectively across a wide temperature range, which is advantageous for various applications, from consumer electronics to industrial systems.
V. Applications of Supercapacitors
Supercapacitors find applications across a diverse range of sectors:
A. Consumer Electronics
In devices like smartphones and laptops, supercapacitors can provide quick bursts of power, enhancing performance during peak usage.
B. Electric Vehicles
Supercapacitors are increasingly used in electric vehicles for regenerative braking systems, allowing for rapid energy recovery and improved efficiency.
C. Renewable Energy Systems
In solar and wind energy systems, supercapacitors can store excess energy generated during peak production times, providing a buffer for energy supply.
D. Industrial Applications
Supercapacitors are used in various industrial applications, including uninterruptible power supplies (UPS) and backup power systems, where reliability and quick response times are critical.
E. Emerging Technologies
As technology advances, supercapacitors are being integrated into new applications, such as smart grids and IoT devices, where efficient energy management is essential.
VI. Advantages and Limitations
A. Advantages of Supercapacitors
1. **Fast Charging and Discharging**: Supercapacitors can be charged and discharged in seconds, making them ideal for applications requiring rapid energy delivery.
2. **Long Cycle Life**: With the ability to withstand millions of cycles, supercapacitors offer a longer lifespan compared to traditional batteries.
3. **Wide Temperature Range**: Their ability to operate effectively in various temperatures makes them versatile for different environments.
B. Limitations
1. **Lower Energy Density Compared to Batteries**: While supercapacitors excel in power density, their energy density is lower than that of batteries, limiting their use in applications requiring long-term energy storage.
2. **Cost Considerations**: The manufacturing costs of high-performance supercapacitors can be higher than traditional batteries, which may hinder widespread adoption.
3. **Environmental Impact**: The production and disposal of certain materials used in supercapacitors can raise environmental concerns, necessitating sustainable practices.
VII. Future Trends and Innovations
A. Research and Development Directions
Ongoing research aims to enhance the energy density and reduce the costs of supercapacitors, making them more competitive with traditional energy storage solutions.
B. Potential for New Materials
Innovations in materials science, such as the development of nanomaterials and advanced composites, hold promise for improving supercapacitor performance.
C. Integration with Other Energy Storage Technologies
The future may see supercapacitors being integrated with batteries and other energy storage technologies to create hybrid systems that leverage the strengths of each.
D. Market Growth and Economic Impact
As the demand for efficient energy storage solutions continues to rise, the supercapacitor market is expected to grow significantly, impacting various industries and driving economic development.
VIII. Conclusion
Supercapacitors represent a vital component of the future energy landscape, offering unique advantages in energy storage and delivery. Their ability to charge and discharge rapidly, coupled with a long cycle life, positions them as a key player in various applications, from consumer electronics to renewable energy systems. As research and development continue to advance, supercapacitors will likely play an increasingly important role in shaping sustainable energy solutions for the future.
IX. References
- Academic Journals
- Industry Reports
- Books and Articles on Energy Storage Technologies
In summary, supercapacitors are not just a niche product; they are a transformative technology that can enhance energy efficiency and sustainability across multiple sectors. Their unique structure and performance characteristics make them an essential part of the evolving energy landscape.